Lens Theory
Lense Theory
Oftentime a user is faced with the question of which lens is best suited for his usecase. In the following the theory of standard lens types is described.
Singlet Lenses
Nomenclature
Fig. 1 is a common diagram used to define quantities for the analysis of a singlet lens. The quantities defined can also be extended to multi-
element systems by procedures outlined in optical design textbooks.
The optical axis (O-O’) of the lens is a line passing through the centers of curvature of the two spherical lens surfaces (centers of curvature not shown).
Ray A is incident from left to right and parallel to the optical axis. Ray A is refracted to point F2 on axis, the back, second, or secondary focal point.
Ray B is incident from right to left and parallel to the optical axis. Ray B is refracted to point F1 on axis, the front, first, or primary focal point.
Suppose the lens is „well-corrected“. That means all rays A parallel to the axis are focused to a single point F2, regardless of their distance from the axis. It is clear then that the family of rays refracted to F2 intersect the family of progenitor rays A in a sphere centered at F2. This sphere, or, in general, surface of intersection of progenitor and refracted rays is called the back, second, or secondary principal surface. The intersection H2 of this surface with the optical axis is called the back, second, or secondary principal point.
Similarly, rays B incident from the right, and their refracted rays impinging on F1, intersect to form a front, first, or primary principal surface, which intersects the optical axis at H1, the front, first, or primary principal point.
In the paraxial approximation, all ray angles are small. This
approximation permits the modelling of the principal surfaces as
principal planes. The distances from the front and back principal points to their respective focal points are equal and given by f , the focal length or effective focal length. The front and back focal distances FFD and BFD, respectively, are measured from the surface intersections V1 and V2 to the respective focii. V1 and V2 are called the front and back vertices.
Singlet Lenses - Quantitative
To calculate these quantities above we need sign conventions.
- Distance increases towards the right.
- A surface has positive curvature if its center of curvature is to the right of the vertex of the surface. In other words, if you have to move in the positive direction from the vertex of a surface to reach its center of curvature, the curvature is positive.
- Angles increase from zero, parallel to the optical axis, to positive values in a counterclockwise direction from the optical axis.
- V1H1 and H2V2 are positive if the principal points are to the right and left, respectively, of their corresponding vertices. Note that the principal points can fall outside of the lens.
The formulas below now permit the calculation of the effective focal length, front and back focal distances, and positions of the principal points of a singlet lens in the paraxial approximation. The distance tc below is the center thickness of the lens V1V2.
Important Quantities for Singlet Lenses Immersed in Air
SYMBOL:
f
Description:
eff
SYMBOL:
BFD
Description:
back focal distance
SYMBOL:
FFD
Description:
front focal distance
SYMBOL:
H2V2
Description:
back principal point to back vertex distance
SYMBOL:
V1H1
Description:
front vertex to front principal point distance
Focal Length Formulas for Simple Singlet Lenses in Air. The Radii are considered positive in the formulas below.
For plano convex, plano concave, equiconvex and equiconcave lenses, we give the focal lengths in the
following table. (Principal plane data may be calculated using the tables above.)

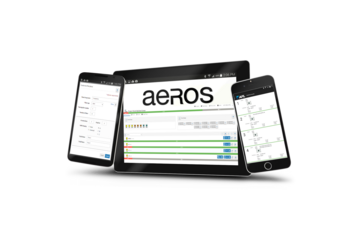
Plano Convex

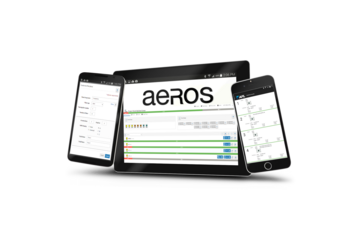
Plano Concave

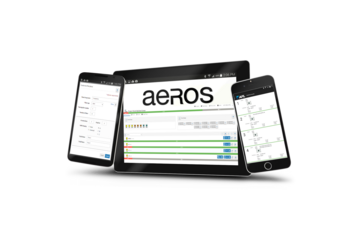
Equiconvex

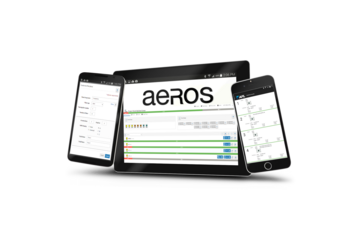
Equiconcave
GATED
Beyond Borders
Performance and Proper Orientation of Singlet Lenses for Minimum Spot Size
High quality singlet lenses are of particular interest in laser focusing and beam handling applications because of their low cost, high damage threshold, and availability of a large variety of standard parts. We offer a large selection of BK7 and UV grade fused silica singlets that can satisfy most monochromatic focusing requirements. Can singlet lenses provide diffraction limited performance in the focusing of collimated beams? Examine the figure below.
This figure is a geometrical ray trace of 25 rays in the focal region of a 100 mm focal length BK7 plano convex lens (n= 1.515). The lens is oriented with the plano surface towards the focus and the origin (0 mm) is at the back vertex (V2) of the lens. The rays were launched parallel to the axis and equally spaced in a region 16 mm above and below the axis in a plane containing the axis (meridional plane). Therefore, the lens is operating at the rather ”fast“ aperture of f/3.125. The marginal rays reach the axis first, in plane MP. The paraxial rays reach the axis last, at the paraxial focal plane PP. The distance from V2 to PP is the back focal distance (BFD) of the 100 mm focal length lens. This can be verified using the formulas previously defined. The distance from PP to MP, here negative, is the longitudinal spherical aberration LAm of the marginal rays. The beam width in the paraxial focal plane PP is the transverse spherical aberration TSAm, determined by the heights of the marginal rays. Notice that the smallest geometrical spot size can be found at plane MS, approximately 3/4 LAm back toward the lens from the paraxial focal plane.
Shape Factor
For singlet lenses, the smallest beam diameter dMS at plane MS can be computed from third order aberration theory. The result is:
dMS = f ∆θ blur
where ∆ blur is the ”full angle angular blur“ of the lens for collimated input light, given by:
∆θ blur = (d0/f)³ [ n² – (2n+1) K + (n+2) K² / n ] / 32(n–1)²
where d0 is the input beam diameter and K is the shape factor of the lens given by:
K = R2 / ( R2 – R1)
Note that the lens orientation is contained in K. The angular blur is plotted as a function of shape factor for three typical glasses used in our singlets.
Geometrical optics angular spot size for spherical singlet lens for indices of refraction typical of fused silica (n = 1.4942 at 280.0 nm), BK7 (n = 1.5205 at 514.5 nm) and SF11 (n = 1.7648 at 800.0 nm). A collimated beam is assumed incident on R1. To use this graph, find the shape factor of the lens using the proper sign conventions for R1 and R2. Read the angular blur, in units where d is the beam diameter and f is the focal length. Now calculate the actual angular blur in radians by multiplying by (d0/f)³. Finally, multiply by the focal length to obtain the spot size in units of length. If the value is much less than the diffraction limit, performance of the lens will be limited by diffraction rather than spherical aberration.
Three important points should be noted:
- The error of misorienting a plano convex lens approximately quadruples the blur.
- Use of a best form lens (shape factor corresponding to the blur minima) for any of the three glasses shown is hardly worth the expense.
- The high index of SF11 significantly reduces singlet aberration.
For this example, (K = 1), we compute a geometrical optics minimum beam size dMS = 226 µm.
LAm is given by:
LAm = -4f² ∆θblur / d0
The minimum spot size occurs at a distance s2 = BFD - 3/4 LAm from the rear vertex of the lens. In this example, LAm = -2.82 mm and s2 = 93.92 mm.
A 226 µm spot size obtained by focusing a 32 mm diameter collimated beam is hardly diffraction limited. We have purposely chosen a highly aberrated situation as an example. The diffraction limited full angle angular blur with aperture limited by the beam diameter d0 is:
∆θ diff = 2.44 l / d0
implying a diffraction limited spot size of:
Δθiff = f ∆θ diff = 2.44 l f / d0
This is the diameter of the first dark ring of the Airy pattern in the focal plane. For the above example,
ddiff = 4.8 µm at a wavelength of 632.8 nm. Comparing the aberration limited geometrical optic spot size
f ∆θ blur to the diffraction limited spot size f ∆θdiff suggests a criterion for diffraction limited operation of a
singlet lens. Compute the focal length as a function beam diameter where
f ∆θblur = f ∆θ diff.
If f ∆θblur > f ∆θdiff the spot size is limited by aberration.
If f ∆qθblur < f ∆θdiff the spot size is essentially diffraction limited.
The curves on the following page depict this computation for properly oriented plano convex BK7 and fused
silica singlets. To use the curves, find the beam diameter and desired focal length point on the graph. If this point is above the curve for intended wavelength, plano convex singlet lens performance is essentially diffraction limited. If this point is on or below curve, consider using a doublet to decrease aberrated spot size.
- In designing optical systems for low divergence laser beams, the paraxial approximation is excellent as far as the positioning of the elements is concerned. However, due to diffraction, the magnifications encountered may be completely different than those calculated from geometric optics. Use the familiar ABCD matrix formalism. If you treat the lenses as thin lenses, use distances between principal points to determine the element positioning. If you use the actual radii of curvature, indices of refraction, and center thicknesses in a more detailed calculation, your calculation will already implicitly take into account the principal planes.
- Formulas for Gaussian beam waists are readily derived using standard ABCD matrix formalism. Do not take the beam diameter results too seriously, however, unless you are working with cw HeNe, ion, Ti:Sapphire, Nd:YAG, or dye lasers with published and verifiable TEMoo waists and locations. In particular, ”near-Gaussian“ pulsed solid state lasers are many times diffraction limited as may be verified by comparing the manufacturers beam diameter and full angle divergence specifications. Single-stage excimer and copper vapor lasers are even more divergent, even when using unstable resonator optics. (Here, the beam divergence actually varies during the pulse, with the brightness increasing with round trip number.) For these lasers, the divergence limited spot size is given by daf · ∆θ, where ∆θ is the measured full angle divergence. It is this spot size that should be computed and compared to the spot size due to spherical aberration in the choice of a focusing lens.
Publication of all information and illustrations has been handled with great care. Nevertheless, errors might be possible. Therefore, no
guarantee or juristic liability whatsoever are granted by the publishers as well as the authors concerning incorrect statements and their
consequences; no liability for damages and/or subsequent damages can be accepted.
Downloads
Beyond Borders
LASER COMPONENTS Nordic - Your competent partner for optical and optoelectronic components in Sweden, Norway, Finland, Denmark, and Iceland.
Welcome to LASER COMPONENTS Nordic AB, your expert for photonics components. Each product in our wide range of detectors, laser diodes, laser modules, optics, fiber optics, and more is worth every Crown (SEK). Our customized solutions cover all conceivable areas of application: from sensor technology to medical technology. You can reach us here:
Box 14001 / Skårs led 3
SE-400 20 Göteborg
Sweden
Phone: +46 (0)31 703 7173
Email: info(at)